In which ontogenetic phase is the bone: attainment, maintenance, or senescence (aged)? It is important to review terms that are used to describe phases of a bone’s ontogeny so that possible genetic, epigenetic, and extra-genetic influences on bone modeling and remodeling can be more precisely considered and discussed.
There is an evolving neology for the traditional use, and present polysemy, of the terms “genetic” and “epigenetic”. For this reason it is wise to avoid using the more conventional dichotomous terms “intrinsic” (i.e., “genetic”) vs. “extrinsic” (i.e., “epigenetic”) influences in skeletal development and adaptation. This is because the concept of “extrinsic” factors must be expanded to include non-heritable “extra-genetic” stimuli (see below). This relatively new usage (Skedros et al., 2007) differs from the ‘conventional’ use of genetic and epigenetic when considering bone adaptation (e.g., see Pearson and Lieberman (2004)). Extra-genetic (environmental) influences that affect bone adaptation include microdamage events and strains that exceed hypothesized thresholds (Skedros et al., 2001) (Fig. 1). These “influences” or “stimuli” are not heritable (in contrast to genetic and epigenetic influences). It is suggested that extra-genetic factors can have important influences on bone’s structural and material organization by activating/modifying bone modeling and remodeling processes. However, it is important to consider that while extra-genetic stimuli may not be heritable, they do act on genetic and cellular machinery that is heritable.
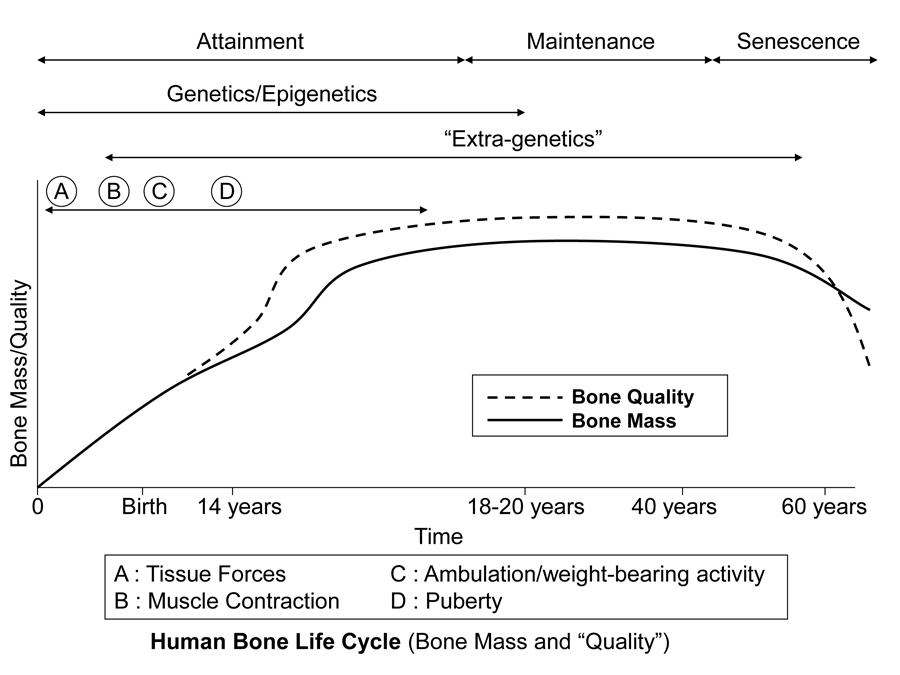
Figure 3 is a diagrammatic depiction of presumed temporal influences of genetic, epigenetic, and extra-genetic factors throughout the attainment, maintenance (from skeletal maturity to non-elderly adult), and senescence phases of skeletal ontogeny. In addition to considering how a bone’s morphology (Table 1) might be influenced by one or more of these factors, it is also important to simultaneously know which phase of ontogeny it is in, or if it is in a transition zone between phases. For example, this is especially important in cases where there could be important aging effects on bone microstructure. Furthermore, modeling activities can be relatively difficult or impossible to evoke — but remodeling activities are not — when the animal is well into, and beyond, the maintenance phase.
Fig. 1 This stylized depiction of ontogenetic changes in bone mass and “quality” (e.g., bone tissue mechanical properties) in humans and is adapted from several sources (Biewener and Bertram 1993; Carter and Beaupré 2001; Kassem et al. 1996). This figure helps to conceptualize the putative shifts in the temporal importance of genetic, epigenetic, and extra-genetic influences, especially with respect to varying histocompositional characteristics within or between bones. In this context, for example, the regional material variations that emerge in the “tension” and “compression” cortices during mid-to-late phases of growth of horse radii (Riggs et al. 1993) and sheep calcanei may serve to enhance the toughness and fatigue resistance for these habitual non-uniform strain environments. From the pre-natal phase and well into the attainment phase, the adaptive growth response of cartilage, or chondral modeling (not discussed in this chapter), has a profound influence on the growth and form of limb bones, especially at their epiphyses where articulations are formed with adjacent bones (Hammond et al. 2010). Shown is also the second bone mass growth spurt that occurs in humans, which has not been demonstrated in any other amniote.
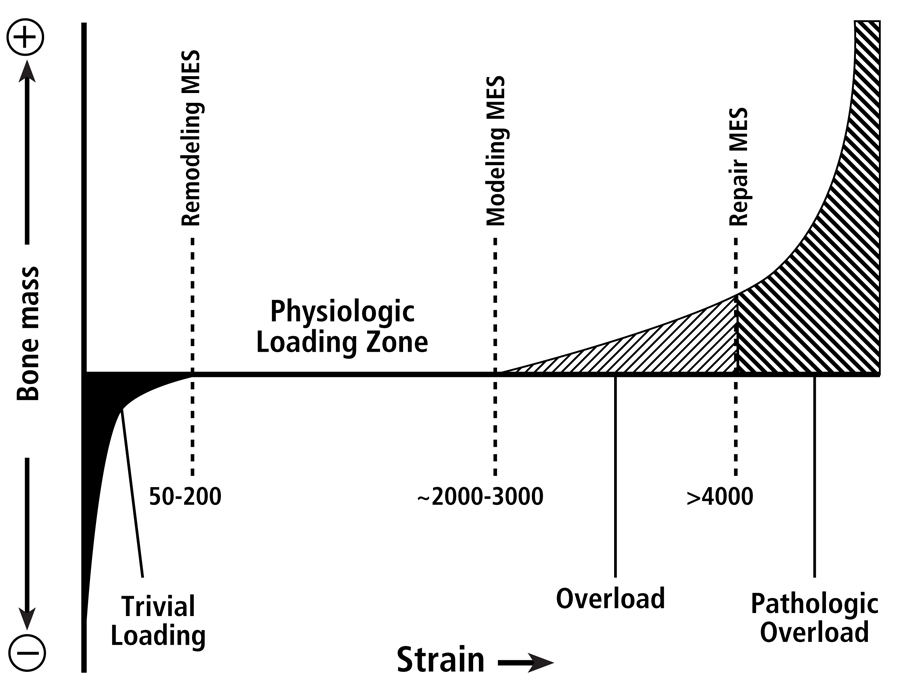
Fig. 2 The four mechanical usage windows or zones, according to the Mechanostat hypothesis as popularized by Harold Frost. This figure emphasizes that there are differences between thresholds for modeling and remodeling activities. Below the minimum effective strain of remodeling (MESr) (low or “trivial” loading zone), strains are low and bone remodeling is activated. Above the remodeling MESr but below the modeling MESm (the physiologic loading zone), remodeling activity is relatively repressed, and is also under the influence of hormonal and metabolic influences. [This physiologic loading zone is analogous to the “lazy” zone described by Carter et al. (1996) and “dead” zone described by Huiskes et al. (1992) (see Burr (1992).] Above the modeling MESm, lamellar bone is gained through increased modeling. Above the repair MESp (pathologic overload zone), new woven bone is added rapidly to bone surfaces; this is neither modeling nor remodeling, but probably represents a repair reaction. There are data suggesting that a site-specific customary strain stimulus exists for each site within the skeleton (even within the same diaphysis), and it is that combination of different strain parameters to which the modeling and remodeling processes are directed (Skerry 2006).
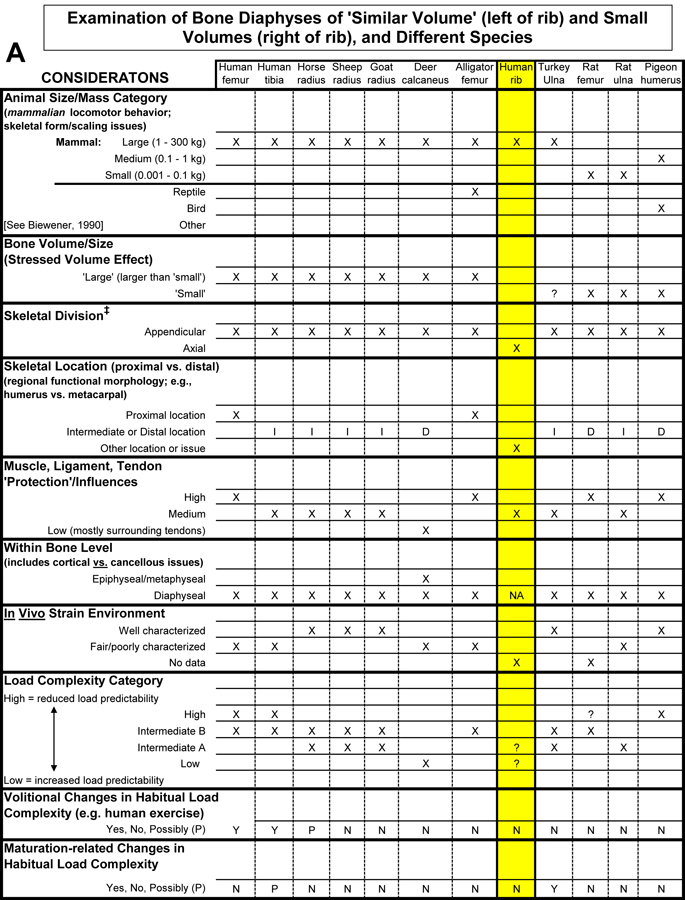
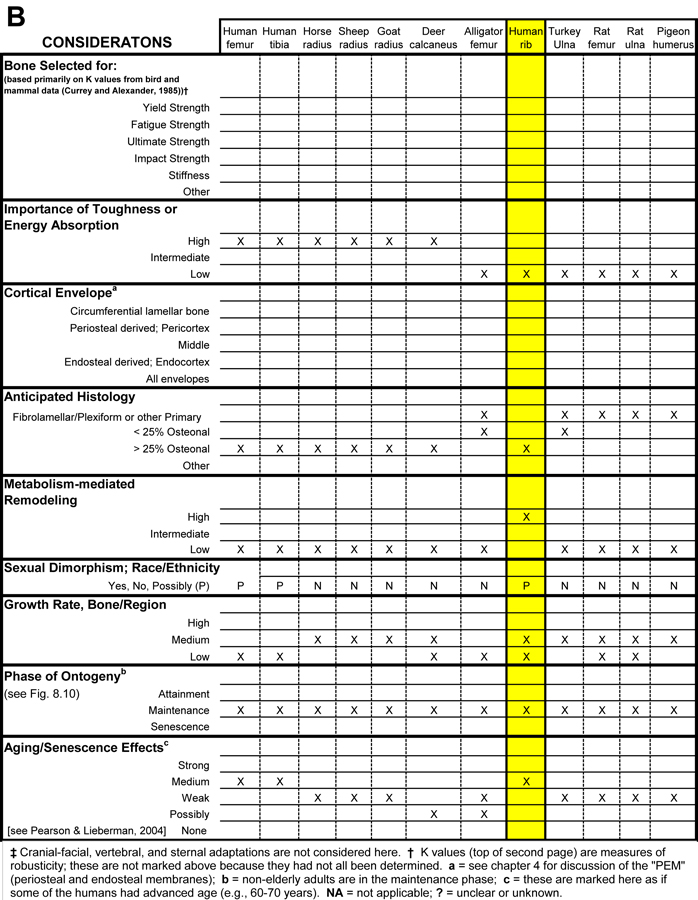
Table 1. Worksheet/checklist of important considerations for interpreting adaptation in the appendicular skeleton.
References
Biewener AA, Bertram JEA (1993) Mechanical loading and bone growth in vivo. In Bone, Volume 7, Bone Growth – B (ed Hall BK), pp. 1-36. Boca Raton, FL: CRC Press.
Burr DB (1992) Orthopedic principles of skeletal growth, modeling and remodeling. In Bone Biodynamics in Orthodontic and Orthopedic Treatment (eds Carlson DS, Goldstein SA), pp. 15-50. Ann Arbor, MI: University of Michigan.
Carter DH, Beaupré GS (2001) Skeletal function and form. Mechanobiology of skeletal development, aging, and regeneration, University of Cambridge, Cambridge, UK.
C
arter DR, van der Meulen MCH, Beaupré GS (1996) Mechanical factors in bone growth and development. Bone, 18, 5S-10S.
Hammond AS, Ning J, Ward CV, Ravosa MJ (2010) Mammalian limb loading and chondral modeling during ontogeny. Anat Rec (Hoboken), 293, 658-70.
Huiskes R, Weinans H, van Rietbergen B (1992) The relationship between stress shielding and bone resorption around total hip stems and the effects of flexible materials. Clin Orthop, 124-34.
Kassem M, Melton LJ, Riggs BL (1996) The Type I Type II mode for involutional osteoporosis. In Osteoporosis (eds Marcus R, Feldman D, Kelsay J), pp. 691-702. New York: Academic Press.
Pearson OM, Lieberman DE (2004) The aging of Wolff’s law: Ontogeny and responses to mechanical loading in cortical bone. Yearbook of Physical Anthropology, 47, 63-99.
Riggs CM, Lanyon LE, Boyde A (1993) Functional associations between collagen fibre orientation and locomotor strain direction in cortical bone of the equine radius. Anat Embryol, 187, 231-238.
Skedros JG, Mason MW, Bloebaum RD (2001) Modeling and remodeling in a developing artiodactyl calcaneus: A model for evaluating Frost’s mechanostat hypothesis and its corollaries. Anat Rec, 263, 167-185.
Skedros JG, Sorenson SM, Hunt KJ, Holyoak JD (2007) Ontogenetic structural and material variations in ovine calcanei: a model for interpreting bone adaptation. Anat Rec, 290, 284-300.
Skerry TM (2006) One mechanostat or many? Modifications of the site-specific response of bone to mechanical loading by nature and nurture. J Musculoskelet Neuronal Interact, 6, 122-7.