The Stressed “Volume Effect”
The stressed “volume effect” helps distinguish fundamental differences in the adaptability of bones from small vs. large animals. The ‘volume effect’ also helps explain why some bones do not form osteons. Material science teaches the crux of the volume effect: larger structures are more prone to failure than small structures. An explanation for this difference is that failures are initiated at flaws in the material of a structure, and larger volumes are more likely to contain a significant flaw (Martin, 2003). In applying this concept to the comparison of a large-volume vs. a small-volume bone made of the same material, Taylor (2000) argues that the larger bone is more prone to fatigue failure than the smaller bone. In this situation, natural selection would favor a mechanism for reducing and repairing the higher probability of flaws in large-volume bones. This seems to be the most compelling and parsimonious explanation for the existence of secondary osteons and why they often do not form (i.e., are not readily used to renew small packets of tissue) in usual physiological/biomechanical circumstances in small animals (e.g., small mammals like mice and rats, and many birds).
This next point is not intuitive ― the volume effect also implies that bones in larger animals cannot withstand as much stress in daily use as bones in smaller animals. Hence, a bone from a large animal would be comparatively weaker, with the stress concentrator effect of osteocyte lacunae also contributing disproportionately. Taylor (2002) suggests that this paradox is resolved in larger animals by enhancing the bone material through histological modifications. Fatigue-related requirements are therefore important for understanding the biomechanical bases of variations in histomorphological characteristics (e.g., osteon population densities, osteon
morphotypes, predominant CFO, and ‘types’ of primary bone) between the different ‘stressed volumes’ of bones of small and large animals. In some cases this interpretation may also help to explain why significant histological (e.g., osteon population density) and/or compositional (e.g., mineral content = ash fraction) differences occur within/between bones of the same limb (e.g., between thin/thick cortices or gracile/robust bones) (Skedros et al., 2004, Skedros et al., 2003, Tommasini et al., 2005). Although, experimental studies have confirmed the basic premise of the volume effect (Bigley et al., 2007, Bigley et al., 2008), some caution must be exercised when drawing comparisons between species that exhibit differences in histology (at least in younger animals). This is because these differences might reflect substantial dissimilarity in bone appositional growth rates, perhaps for precocial vs. altricial ambulation.
Why consider the stressed volume effect in physical anthropology studies?
This concept is very important because a question that all enthusiasts of bone adaptation eventually ponder is: “Why do secondary osteons form in some bones but not in others?” What then follows is typically a discussion of the relative importance of osteonal remodeling in terms of microdamage repair vs. metabolism (e.g., making calcium available for metabolic demands such as dietary stress and lactation). Therefore, the volume effect helps in forming a phylogenetic-, ontogenetic-, and biomechanical-based framework for explaining why the capacity for osteon-based tissue renewal never, or nearly never, forms in some bones.
The volume effect also helps provide the intellectual framework for identifying the constraints inherent in comparing or extrapolating adaptations and adaptability between osteonal bones (typically larger) and non-osteonal bones (typically smaller). For example, it helps to avoid the assumption that modeling/remodeling ‘rules’ generally apply to all bones of vertebrates (see definitions of modeling and remodeling in the Appendix). For example, there may be little value in attempting to extrapolate the adaptability of a rat ulna to a human tibia (Tami et al., 2003). This is especially true in terms of many morphological characteristics because relatively small volume bones are constrained in their potential for histological adaptation. In contrast to the large-volume human tibia (Ebacher et al., 2007), the modeling process of the rat ulna is sufficient for affecting all mechanical adaptations for physiological and supra-physiological loading. If a bone does not exhibit even modest osteon formation, then this could invalidate comparisons of its modeling-mediated adaptations to a bone that models and remodels. For example, compare and contrast the studies of small-volume bones by Schneider et al. (2007), Tami et al. (2003), Carlson and Judex (2007), Kumar et al. (2010), Pinto et al. (2010), and Wallace et al. (2010) in terms of how they (or you might) consider or extrapolate their results to be functional adaptation of hominid limb bones.
Exceptions to the rule and the human bias.
Caution must be exercised when considering the volume effect in some cases because there are examples in larger animals that have greater remodeling in their smaller-volume bones. For example, human metacarpals have been shown to be highly remodeled (Coutelier, 1976, Koltze 1951). Figure 1 also shows that the smallest bone in the deer forelimb skeleton is the most highly remodeled (this observation led to the inclusion of the ‘proximal vs. distal bone’ consideration in Table 1. These examples show that unresolved species-related or other less-than-simple biomechanical issues are at play (Lieberman et al., 2003, Drapeau and Streeter, 2006, Skedros et al., 2003).
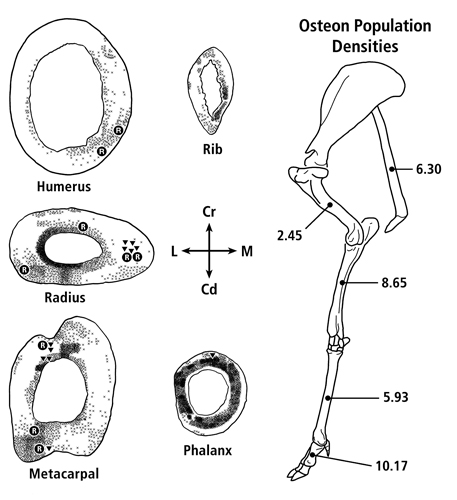
Fig. 1 At left are transverse sections of the mid-diaphyses of the forelimb bones from one of the mature mule deer examined by Skedros et al. (2003b). X = secondary osteons, R = resorption spaces, and triangles (▼) = newly forming osteons. Secondary osteons typically occupy approximately 30% or less of the mid-diaphyseal cortex of the principal metacarpal, radius, and humerus. At right is the side view of the forelimb skeleton of an adult Rocky Mountain mule deer showing the averaged values of osteon population densities (no./mm2) at mid-diaphysis from 11 adult animals.
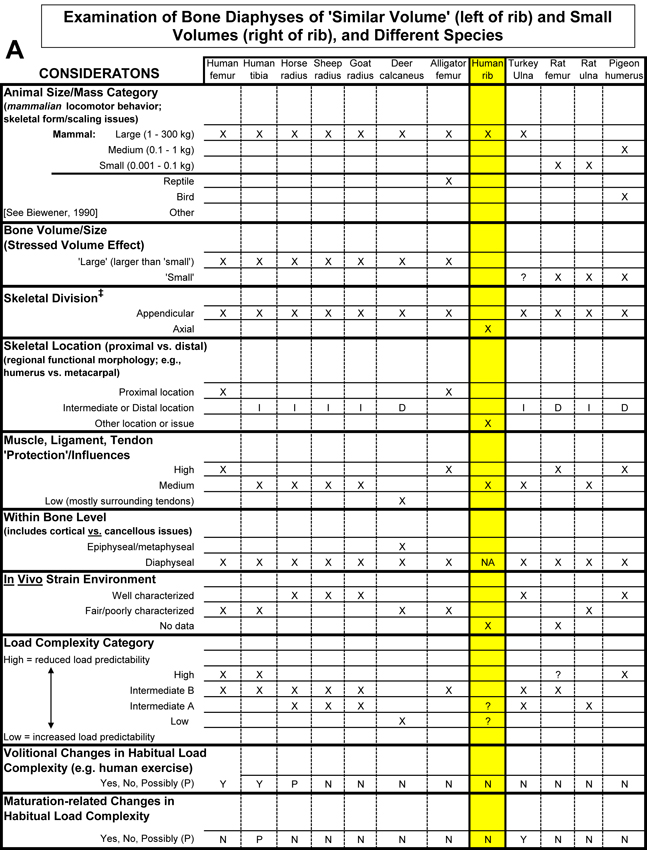
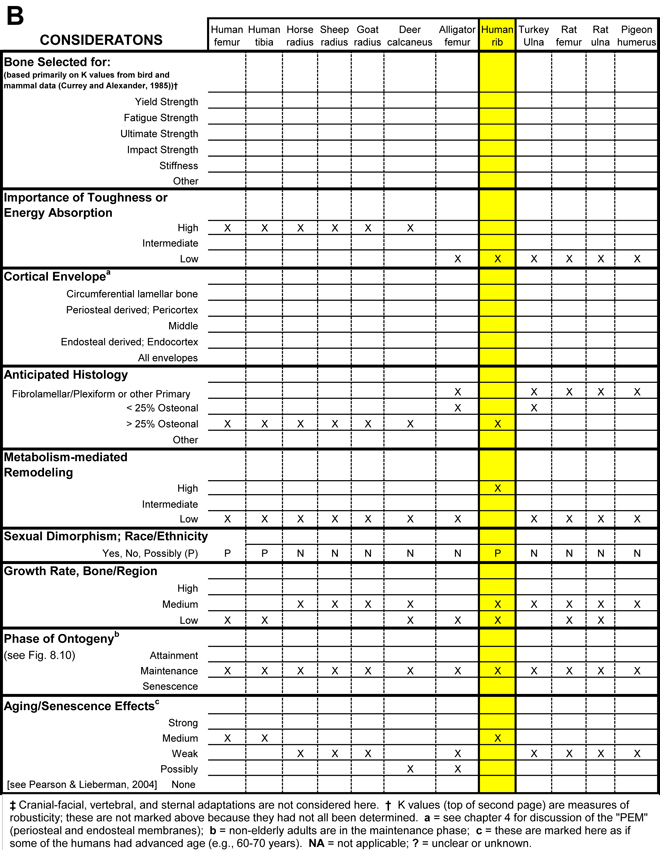
Table 1 Worksheet/checklist of important considerations for interpreting adaptation in the appendicular skeleton.
What also makes it difficult to apply the volume effect in some comparative cases is what can be called the dual ‘influences’ of the human bias and the altricial/precocial growth or ambulation distinction. The human bias is the common idea that osteonal bone is somehow better because it is prevalent in humans, even at a relatively young age. But this view must be reconciled with the predominance of primary bone (fibrolamellar/plexiform) that works quite well in many other mammals, especially earlier in their growth (Currey, 2003). In order to avoid the pitfalls of these biases, it is useful to determine if the growth, ambulation, and/or other ambulation-related activity (e.g., flight in birds) of the bone or limb are altricial or precocial (see Skedros (2012) for more discussion). Table 1 lists some additional issues (e.g., body mass and skeletal scaling relationships) that can help one remember that there are other potentially important considerations when comparing small-volume bones to large-volume bones.
References
Bigley RF, Gibeling JC, Stover SM, Hazelwood SJ, Fyhrie DP, Martin RB (2007) Volume effects on fatigue life of equine cortical bone. J Biomech, 40, 3548-54.
Bigley RF, Gibeling JC, Stover SM, Hazelwood SJ, Fyhrie DP, Martin RB (2008) Volume effects on yield strength of equine cortical bone. J Mech Behav Biomed Mater, 1, 295-302.
Britz HM, Thomas CD, Clement JG, Cooper DM (2009) The relation of femoral osteon geometry to age, sex, height and weight. Bone, 45, 77-83.
Carlson KJ, Judex S (2007) Increased non-linear locomotion alters diaphyseal bone shape. J Exp Biol, 210, 3117-25.
Coutelier L (1976) Le remaniement interne de l’os compact chez l’enfent (The internal remodeling of compact bone in children). Bulletin de l’ association des anatomistes (Bull Assoc Anat), 60, 95-110.
Currey JD (2003) The many adaptations of bone. J Biomech, 36, 1487-95.
Drapeau MS, Streeter MA (2006) Modeling and remodeling responses to normal loading in the human lower limb. Am J Phys Anthropol, 129, 403-9.
Ebacher V, Tang C, McKay H, Oxland TR, Guy P, Wang R (2007) Strain redistribution and cracking behavior of human bone during bending. Bone, 40, 1265-75.
Koltze H (1951) Studie zur äusseren Form der Osteone. Zeitschrift fuer Anatomie und Entwicklungsgeschichte, 115, 585-596.
Kumar NC, Dantzig JA, Jasiuk IM, Robling AG, Turner CH (2010) Numerical modeling of long bone adaptation due to mechanical loading: correlation with experiments. Annals of Biomedical Engineering.
Lieberman DE, Pearson OM, Polk JD, Demes B, Crompton AW (2003) Optimization of bone growth and remodeling in response to loading in tapered mammalian limbs. J Exp Biol, 206, 3125-3138.
Martin RB (2003) Fatigue damage, remodeling, and the minimization of skeletal weight. J Theor Biol, 220, 271-6.
O’Brien FJ, Taylor D, Lee TC (2002) An improved labelling technique for monitoring microcrack growth in compact bone. J Biomech, 35, 523-6.
Paine RR, Godfrey LR (1997) The scaling of skeletal microanatomy in non-human primates. J. Zool. Lond., 241, 803-821.
Pinto M, Jepsen KJ, Terranova CJ, Buffenstein R (2010) Lack of sexual dimorphism in femora of the eusocial and hypogonadic naked mole-rat: a novel animal model for the study of delayed puberty on the skeletal system. Bone, 46, 112-20.
Schneider P, Stauber M, Voide R, Stampanoni M, Donahue LR, Muller R (2007) Ultrastructural properties in cortical bone vary greatly in two inbred strains of mice as assessed by synchrotron light based micro- and nano-CT. J Bone Miner Res, 22, 1557-70.
Skedros JG (2012) Interpreting load history in limb-bone diaphyses: Important considerations and their biomechanical foundations. In Bone Histology: An Anthropological Perspective (eds Crowder C, Stout S), pp. 153-220. CRC Press.
Skedros JG, Grunander TR, Hamrick MW (2005) Spatial distribution of osteocyte lacunae in equine radii and third metacarpals: considerations for cellular communication, microdamage detection and metabolism. Cells, Tissues, Organs, 180, 215-236.
Skedros JG, Hunt KJ, Bloebaum RD (2004) Relationships of loading history and structural and material characteristics of bone: Development of the mule deer calcaneus. J Morphol, 259, 281-307.
Skedros JG, Sybrowsky CL, Parry TR, Bloebaum RD (2003) Regional differences in cortical bone organization and microdamage prevalence in Rocky Mountain mule deer. Anat Rec, 274A, 837-850.
Tami AE, Nasser P, Schaffler MB, Knothe Tate ML (2003) Noninvasive fatigue fracture model of the rat ulna. J Orthop Res, 21, 1018-24.
Taylor D (2000) Scaling effects in the fatigue strength of bones from different animals. J Theor Biol, 206, 299-306.
Tommasini SM, Nasser P, Schaffler MB, Jepsen KJ (2005) Relationship between bone morphology and bone quality in male tibias: implications for stress fracture risk. J Bone Miner Res, 20, 1372-80.
Wallace IJ, Middleton KM, Lublinsky S, et al. (2010) Functional significance of genetic variation underlying limb bone diaphyseal structure. Am J Phys Anthropol.