Changes in strain distribution caused by changes in load predictability can lead to stress fractures: An example in thoroughbred horses.
The role of osteon morphotypes and/or predominant collagen fiber orientation (a hypothesis).
We consider the study by John Bertram and Andrew Biewener published in 1988 in the Journal of Theoretical Biology as one of the most important papers about bone adaptation/biology from the 20th century. This paper is entitled: Bone curvature: sacrificing strength for load predictability? Here is the abstract of that study:
Nearly all long bones of terrestrial mammals that have been studied are loaded in bending. Yet bending requires greater bone mass than axial compression for effective support of equivalent static loads. Most long bones, in fact, are curved along their length; their curvature augmenting rather than diminishing stresses developed due to bending. The most “efficient” design of a bone (maximal strength per unit mass) should be a form which is straight and resists axial compression. Bone curvature and the bending developed in the long bones of most species studied, therefore, poses a paradox in design. However, under natural conditions an animal’s skeleton must support a range of dynamic loads that vary in both direction and magnitude. Thus, improved predictability of dynamic loading should represent an important feature in the design of the bone, in addition to its absolute strength. We present an explanation of long bone curvature, based on the conditions of stability for bending vs. axial compression in a column that describes this apparent design paradox as a mechanism for improving the predictability of loading direction (and, consequently, the pattern of stresses within the bone). Our hypothesis argues that in order to understand the design “effectiveness” of long bone shape the role of the bone as a structural unit must be redefined to one in which bone strength is optimized concurrently with loading predictability. In agreement with our hypothesis, bone curvature appears to meet this requirement.
Our ‘new idea’ regarding stress fractures involves a change in the direction of loading that results in: (1) a change in the direction of the predictable loads (hence the location of the neutral axis, where shear strains are high, changes), or (2) there is a change from load predictability to load unpredictability. In other words we hypothesize that, in many cases when stress fractures occur, not only is there newly repetitive (and often higher stress) loading of the involved bone(s) but also the strain distribution changes in a way that: (1) the bone is not microstructurally/nanostructually adapted, and (2) does not have time to adequately adapt to the new strain milieu.
In contrast to load predictability, we argue that load unpredictability would not allow for microstructural toughening via osteonal remodeling; in some cases this could lead to catastrophic failure. A poignant example of this is the explanation for why the majority of stress fractures in the equine third metacarpals occur in the dorsal-lateral cortex. Nunamaker (2001) has suggested that the shift from prevalent tension in the dorsal cortex during race training at lower speeds to compression at higher racing speeds may provide an explanation for these fractures in thoroughbred horses. He suggests that tension-related adaptations produced by modeling and remodeling activities (see footnote) would not be expected to accommodate compression strains produced at greater speeds: ‘It seems obvious that bone that models and remodels for tensile forces on the dorsal aspect of MCIII [third metacarpal] will be poorly adapted for the large compressive strains that are seen during racing’ [p. 213 (Nunamaker, 2001)]. In turn, when the tension-adapted dorsal cortex receives more prevalent, high magnitude compression strains microcrack formation is enhanced (Fig. 1). This probability is supported by Reilly and Currey (2000) who showed that microdamage formed in compression is highly detrimental to tensile mechanical properties. In tension vs. compression, microdamage not only occurs at different thresholds (more readily forms in tension), but can exhibit different morphologic characteristics (e.g. length, shape, and orientation) (Reilly et al., 1997; Boyce et al., 1998; Muir et al., 1999; Reilly and Currey, 1999; Joo et al., 2004; George and Vashishth, 2005)
Figure 1
In third metacarpals from horses that run at high speeds, the probability that microdamage formation might also occur at different strain thresholds for different regions might be especially important for understanding the material organization in the dorsal-lateral region (e.g., increased prevalence of tension- and shear-adapted osteon morphotypes) since it experiences a greater range of strain by being loaded in tension and compression when compared to the palmar-medial and dorsal-medial cortices (Skedros et al., 1996; Skedros et al., 2006; Skedros et al., 2009). The prevalence of shear strains, which can be more deleterious to mechanical properties than tensile strains (Fig. 2), probably increases in the vicinity of the dorsal-lateral cortex during racing because the neutral axis (where shear strains tend to be greatest) traverses this region at these speeds. In view of these possibilities, an equine third metacarpal that is sufficiently exposed to sub-racing speeds would be expected to become adapted primarily to tension and shear in the dorsal/lateral cortices. However, if higher speeds are acutely and more consistently sustained, the dorsal/lateral cortices of the third metacarpal may be exposed to high-magnitude compression for which it has not microstructurally/ nanostructurally adapted.
Footnote 1. In addition to remodeling-mediated adaptation, adaptive modeling-mediated adjustments in the cross-section shape of the third metacarpals can occur in racing Thoroughbreds. This is possible in racing Thoroughbreds because they are skeletally immature (Nunamaker et al., 1989); hence, they still have the capacity to activate sub-periosteal/sub-endosteal bone growth.
of the third metacarpal may be exposed to high-magnitude compression for which it has not microstructurally/ nanostructurally adapted.
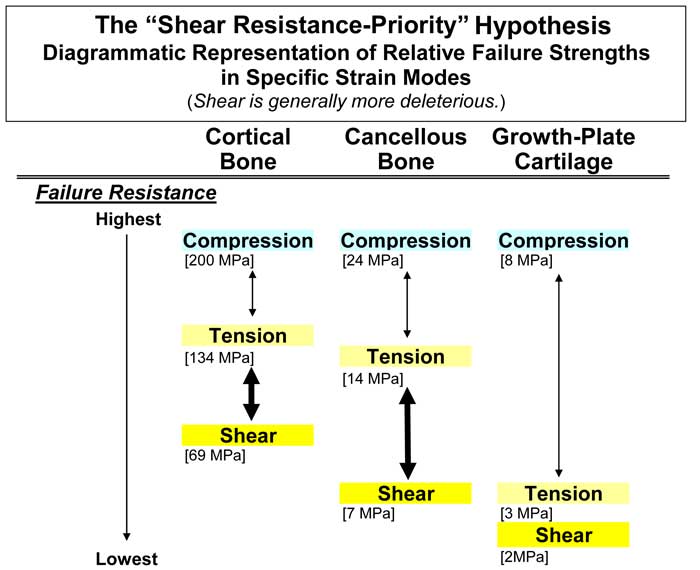
Figure 2
Histological details of the “new idea” with respect to the formation of stress fractures
Experimental studies suggest that different osteon morphotypes modulate and optimize energy absorption for the locally prevalent strain mode (Hiller et al., 2003; Bigley et al., 2006). There are data suggesting that this can also be achieved by regional variations in predominant collagen fiber orientation (hence can be achieved independent of the presence of secondary osteons) (Skedros et al., 2006; Skedros et al., 2014) (see section of this website on “Collagen Fiber Orientation and Osteon Morphotypes”). In habitual “tension regions” and “compression regions”, the natural accumulation of strain-mode-specific microdamage likely also evokes the formation of specific secondary osteon morphotypes. Over time (months/years), cortical regions can become highly populated with different osteon morphotypes (Skedros et al., 2009; Skedros et al., 2011). If the natural remodeling/repair process simply cannot keep pace with the microdamage burden or is otherwise perturbed (e.g., bisphosphonate treatment), then in some regions of the bone non-specific microdamage can accumulate. In these regions we hypothesize that the osteons are not: (1) adequately adapted for the new ‘types’ of microdamage (e.g., regions with “tension morphotypes” now receive compression-type microdamage), (2) shear strains become higher and/or more prevalent in regions that otherwise were microstructurally adapted for habitual tension or compression, and (3) are not able to form quickly enough to produce a sufficient population of ‘beneficial’ osteon morphotypes (i.e., these osteons are beneficial because they are relatively specifically adapted for the prevailing strain mode of the new strain milieu). This set of circumstances is substantially more deleterious than the simple accumulation of microcracks (Reilly and Currey, 1999).
We hypothesize that this scenario is occurring in the proximal femoral diaphysis in some patients who have received pharmacological treatment with bisphosphonates, which, when coupled with the high medial-lateral bending moment in this region, makes it prone to atypical fractures when the remodeling process is perturbed. Could the idea of an activity-induced shifting neutral axis also help to better explain the occurrence of stress fractures in human military recruits or in human femoral neck fragility fractures (Mayhew et al., 2005)? See our abstract that considers this idea in the context of atypical femur fractures that are associated with the use of bisphosphonates for osteoporosis (Skedros et al., 2012) (this study is abstract #98 on this website).
References
Bertram JE, Biewener AA. 1988. Bone curvature: sacrificing strength for load predictability? J Theor Biol 131:75-92.
Bigley RF, Griffin LV, Christensen L, Vandenbosch R. 2006. Osteonal interfacial strength and histomorphometry of equine cortical bone. J Biomech 39:1629-1640.
Boyce TM, Fyhrie DP, Glotkowski MC, Radin EL, Schaffler MB. 1998. Damage type and strain mode associations in human compact bone bending fatigue. J. Orthop. Res. 16:322-329.
George WT, Vashishth D. 2005. Damage mechanisms and failure modes of cortical bone under components of physiological loading. J Orthop Res 23:1047-1053.
Hiller LP, Stover SM, Gibson VA, Gibeling JC, Prater CS, Hazelwood SJ, Yeh OC, Martin RB. 2003. Osteon pullout in the equine third metacarpal bone: Effects of ex vivo fatigue. J Orthop Res 21:481-488.
Joo W, Jepsen KJ, Davy DT. 2004. Complex cross-modal effects of damage on cortical bone properties. Trans Orthop Res Soc 29:515.
Mayhew PM, Thomas CD, Clement JG, Loveridge N, Beck TJ, Bonfield W, Burgoyne CJ, Reeve J. 2005. Relation between age, femoral neck cortical stability, and hip fracture risk. Lancet 366:129-135.
Muir P, Johnson KA, Ruaux-Mason CP. 1999. In vivo matrix microdamage in a naturally occurring canine fatigue fracture. Bone 25:571-576.
Nunamaker D. 2001. Bucked shins in horses. In: Burr DB, Milgrom C, editors. Musculoskeletal Fatigue and Stress Fractures. Boca Raton, Florida: CRC Press LLC. p 203-219.
Nunamaker DM, Butterweck DM, Provost MT. 1989. Some geometric properties of the third metacarpal bone: A comparison between the thoroughbred and standardbred racehorse. J Biomech 22:129-134.
Reilly GC, Currey JD. 1999. The development of microcracking and failure in bone depends on the loading mode to which it is adapted. J Exp Biol 202:543-552.
Reilly GC, Currey JD. 2000. The effects of damage and microcracking on the impact strength of bone. J Biomech 33:337-343.
Reilly GC, Currey JD, Goodship AE. 1997. Exercise of young thoroughbred horses increases impact strength of the third metacarpal bone. J Orthop Res 15:862-868.
Skedros JG, Dayton MR, Sybrowsky CL, Bloebaum RD, Bachus K. 2006. The influence of collagen fiber orientation and other histocompositional characteristics on the mechanical properties of equine cortical bone. J Exp Biol 209:3025-3042.
Skedros JG, Keenan KE, Halley JA, Knight AN, Bloebaum RD. 2012. Osteon morphotypes and predominant collagen fiber orientation are adaptations for habitual medial-lateral bending in the human proximal diaphysis: Implications for understanding the etiology of atypical fractures. 58th Annual Meeting of the Orthopaedic Research Society 37:1512.
Skedros JG, Kiser CJ, Keenan KE, Thomas SC. 2011. Analysis of osteon morphotype scoring schemes for interpreting load history: evaluation in the chimpanzee femur. J Anat 218:480-499.
Skedros JG, Mason MW, Nelson MC, Bloebaum RD. 1996. Evidence of structural and material adaptation to specific strain features in cortical bone. Anat Rec 246:47-63.
Skedros JG, Mears CS, Skedros GA, Henrie TR, Litton SM, Keenan KE. 2014. The mechanical relevance of secondary osteon “morphotypes”: Implications for accommodating non-uniform strains and the etiology of stress fractures. 60th Annual Meeting of the Orthopaedic Research Society:1293-1295.
Skedros JG, Mendenhall SD, Kiser CJ, Winet H. 2009. Interpreting cortical bone adaptation and load history by quantifying osteon morphotypes in circularly polarized light images. Bone 44:392-403.